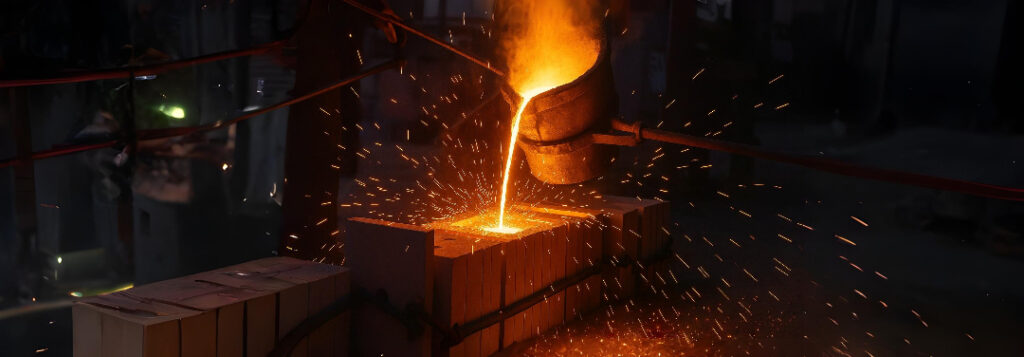
Die Casting Melt Quality Guide
Introduction
Die casting melt quality determines whether a shot emerges as a flawless structural component or an expensive piece of scrap. Hidden inclusions, trapped gases, and turbulent flow can all turn molten metal into a liability. Manufacturers who tame these variables gain leaner cycles, tighter tolerances, and higher customer trust. This article explores what goes wrong in the ladle, why it matters on the balance sheet, and how modern tools allow real‑time control. You will learn the root causes of porosity, practical treatment methods, digital monitoring strategies, smart gating design, and the future of data‑driven foundries.
Inside the Melt: Common Defects
Molten aluminum or magnesium should look like a clean mirror, yet microscopic oxide films often lurk beneath the surface. Hydrogen dissolves quickly at furnace temperature and expands into blisters as metal solidifies. Silicon flakes, iron‑rich intermetallics, and dross fragments add further obstacles to strength. Each defect reduces fatigue life and opens pathways for corrosion. Foundries monitor inclusion area density and gas content because these numbers forecast the final CT scan results. Consistent sampling, reduced pressure testing, and density index measurements create a feedback loop that guides furnace practice and alloy selection. When operators see rising hydrogen parts per million, they can adjust flux additions and crucible atmospheres before defects form.
The Business Case for Superior Melt
Poor die casting melt quality threatens profit on multiple fronts. Scrap consumes energy twice and steals machine hours from good parts. Rejected lots erode customer confidence and invite penalties. Conversely, clean metal lifts tensile strength by double‑digit percentages and enables lightweight designs that win new contracts. Insurance costs fall because fewer claims trace back to porous components. Lean melt practices shorten cycle times as vacuum venting and pressure holding become easier to optimize. Management dashboards now correlate quality metrics with real‑time financial impact, turning melt cleanliness from a foundry concern into a boardroom priority. Investing in degassing, filtration, and training often pays back within a single fiscal year.
Modern Treatment Technologies
Rotary degassers remain the backbone of hydrogen control, but new rotor geometries boost bubble surface area while cutting dross generation. Ceramic foam filters with engineered pore sizes remove oxide bifilms down to tens of nanometres without throttling flow. Fluxing tablets release exothermic heat that drives wetting agents through the melt and lifts inclusions to the surface. Inline grain refiners add titanium and boron just moments before the shot to avoid fade. Vacuum‑assisted high‑pressure die casting (HPDC) extracts residual gases and delivers near‑forge properties. Crucible lids with inert gas blankets stop atmospheric moisture from entering the bath, while laser temperature probes reduce superheat variation to below ±5 °C. Each technique addresses a different segment of the defect spectrum, and their combined effect delivers a dense, consistent microstructure.
Real‑Time Monitoring and Testing
Industry 4.0 has reached the furnace floor. Connected sensors stream temperature, hydrogen ppm, and vibration data to cloud dashboards. Artificial intelligence spots patterns that human eyes miss, predicting inclusion spikes hours in advance. Low‑cost spectrometers mounted on the launder measure alloy chemistry without pausing production. When readings drift, automated dosing stations correct magnesium or strontium content within seconds. Vision systems inspect every casting as it leaves the die and feed defect maps back into gating simulations. This closed loop shortens the time from problem detection to corrective action and transforms melt quality from a periodic check into a live control variable.
Gating and Process Design
Even pristine metal can fail if the gating system folds air into the cavity. Designers achieve laminar flow by using wide sprues, tapered runners, and bottom fills. Simulation software visualizes turbulence and oxide travel, allowing rapid virtual iterations. A gentle initial gate velocity below 0.5 m s‑1 prevents surface film entrainment, while vacuum vents extract the last traces of gas just before solidification. Flow‑straightening ceramic inserts break vortices and align the melt front. When geometry forces high speeds, shot profiles ramp in two stages to avoid impact shock at the die face. Good gating choices therefore extend tool life and preserve the gains made in the furnace.
Future Trends in Melt Quality Control
The next wave of automation will embed digital twins inside every melting cell. Real‑time data streams will update thermodynamic models that suggest flux dosage, degassing time, and optimal shot parameters. Energy‑efficient induction furnaces paired with closed‑loop aluminum recycling will shrink carbon footprints without sacrificing throughput. Start‑ups are already integrating compact CT scanners inline, providing feedback within minutes rather than hours. As electric vehicle platforms demand larger, thinner, one‑piece castings, melt quality tolerances will tighten further. Foundries that master data analytics and sustainable practice will secure their place in the global supply chain.
Conclusion
Melt quality decides the success of every die casting project. By understanding defect mechanisms, treating the melt with modern technology, using live monitoring, and designing gates for smooth flow, manufacturers can slash porosity and raise mechanical performance. Financial gains follow as scrap drops, cycle times shorten, and customer satisfaction rises. The journey toward smart, sustainable foundries begins inside the crucible; keep the metal clean and the data flowing, and every shot will meet or exceed spec on the first attempt.